Join us as we discover the history and the evolution of the battery.
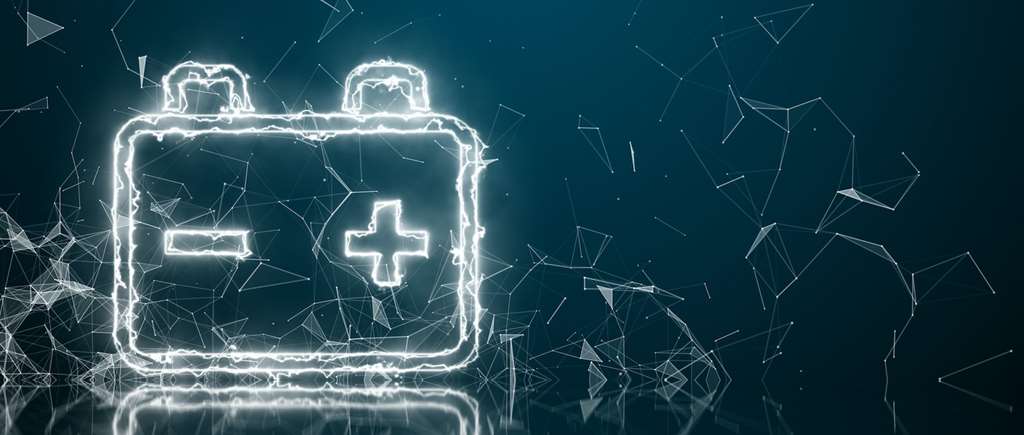
1. The Evolution of Batteries: From Generation to Storage
To appreciate the development of the battery, it is useful to provide you some perspective by first recognising the development of the primary purpose of its invention - that is, the generation and storage of electricity.
2. History of Electricity
Electricity is surely one of the most important discoveries in the last 400 years. It’s actually been around longer than that, though it was never really fully harnessed until much more recently. Examples of electrical current generation have been uncovered dating back 2000 years ago and earlier.
In Baghdad, they unearthed a discovery known as the Parthian battery. It consisted of a clay jar filled with a vinegar solution into which an iron rod surrounded by a copper cylinder was placed. It is believed capable of generating 1.1 to 2.0 volts.
One of the earliest methods of generating electricity in more modern times was by generation of a static charge. Otto von Guericke built an electrical machine in 1660 using a sulfur globe, which when turned and rubbed, attracted feathers and pieces of paper. Guericke proved that the generated sparks were electrical in nature.
In 1744, Ewald Georg von Kleist developed the so-called Leyden jar. It stored static charge in a glass jar lined on both the inside and outside of the container with metallic foil. The “electric pistol” invented by Alessandro Volta was the first known practical use of electricity.
In 1791, Luigi Galvani discovered that when contacted by a metallic object, a frog’s muscles would involuntarily contract. This phenomenon became known as “animal electricity.” Inspired by this work, Volta initiated experiments using zinc, lead, tin, and iron as the cathode and copper, silver, gold, and graphite as the anode. This became known as galvanic electricity, and interest in it quickly spread.
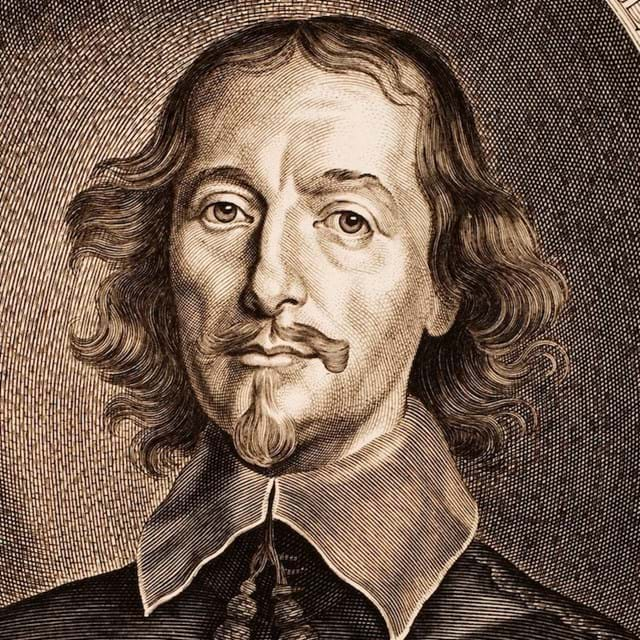
Otto von Guericke generated static charge as early as 1660.
3. Electricity Through Magnetism
In 1820, André-Marie Ampère noted that magnetic forces of attraction and repulsion occurred in wires made to carry an electric current. Michael Faraday demonstrated in 1831 how a copper disc provided a constant electricity flow while revolving in a strong magnetic field. Faraday, with Humphry Davy and his research team, were able to generate a continuous electrical force, provided that the movement between a coil and magnet was uninterrupted. This led to the birth of the electric generator. Generation of electricity no longer depended on a “battery!”
Transformers were developed which converted alternating current (AC) to any voltage. The foundation of electromagnetism, which Faraday’s law is based on, was established in 1833. From an understanding of the relationship with magnetism, large generators were built that were capable of producing a steady flow of electricity. Electric motors soon followed that enabled mechanical movement, and Thomas Edison’s light bulb came to the forefront for brightening a dark world.
Alternating Current Implemented
The earliest electrical plants produced direct current (DC). Unfortunately, the distribution of DC electricity was limited to about 3 km from the generator. Around 1886, Nikola Tesla (1856–1943), created the AC transmission system. The Niagara Falls Power Company (NFPC), with Tesla as a consultant, built the first multi-phase AC system, delivering electricity 20 kilometres away.
In a dazzling display, AC power lit up the Chicago World Fair in 1893. George Westinghouse built three large generators to transform energy from the Niagara Falls to electricity. Tesla later developed three-phase AC technology which enabled the transmission of electric power over great distances economically. Electricity was thus made widely available to industry and consumers.
4. Early Batteries
Volta discovered in 1800 that certain fluids generated a continuous flow of electrical power when used as a conductor. This led to the invention of the first voltaic cell, more commonly known as the battery.
In 1800, Sir Humphry Davy began testing the chemical effects of electricity which led to production and installation of the world’s largest and most powerful electric battery at the Royal Institution of London. Connecting the battery to charcoal electrodes produced the first example of the electric light and was described by observers as “the most brilliant ascending arch of light ever seen.”
William Cruickshank designed the first mass-produced battery in 1802. Its design was a long rectangular wooden box containing square sheets of copper and zinc soldered together. Channels in the box held the metal plates in place. The sealed box was filled with watered down acid serving as the electrolyte. This closely resembled the construction of the flooded lead-acid battery of today.
5. The Invention of the Recheargable Battery
In 1836, John F. Daniell developed a battery which produced a steadier current than earlier attempts to store electrical energy. Then Gaston Planté, in 1859, invented what is widely considered to be the first rechargeable battery. The battery was based on lead-acid, a system that is still in use today. His battery consisted of two strips of tape layered between two lead plates which were rolled into a cylindrical shape. It could be repeatedly charged and discharged in dilute sulfuric acid (electrolyte). The positive electrode was lead dioxide, and the negative electrode was composed of lead active material.
In 1899, Waldemar Jungner invented the nickel-cadmium (NiCd) battery. It employed nickel as the cathode and cadmium as the anode. Its widespread use was limited by relatively high material cost compared to lead. In 1932, Schlecht and Ackermann improved the longevity of the NiCd battery, and in 1947 Georg Neumann succeeded in sealing the cell further improving the NiCd battery, making it a more viable technology.
Alternative Small Device Battery Advancement
The nickel-cadmium (NiCd) battery became the only rechargeable battery for portable applications for many years. However, in the 1990s environmentalists in Europe grew concerned about the harm incurred when NiCd batteries were carelessly disposed. Their sale became restricted by legislation in 2006 in the European Union. Only specialty industrial uses for which there were no viable replacements were exempted from the new rules. Nickel-metal-hydride (NiMH) batteries were later developed and became a more environmentally friendly alternative similar in practical use characteristics to the NiCd battery.
Commercialised by Sony in 1991, lithium-based batteries are the current focus of battery technology development. They are used in a number of applications including portable personal computers, cellular phones, power tools, digital cameras and many other applications. The technology is also the current state of the art for powering electric vehicles. The lithium-ion battery has several benefits, most notably its high energy density, ease of charging and low maintenance requirements.
6. Lead Acid Battery Advances
The development of the lead-acid battery has come some way since Gaston Planté’s raw invention in 1859. Progress in Planté’s design saw the inclusion of multiple cells composed of alternating negative and positive plates suspended in electrolyte with wooden walls between the cells. By 1910, lead-acid batteries were made by using asphalt-coated and sealed wooden containers, thick electrode plates, wooden cell separators between the negative and positive plates and connections between cells made through the cover using heavy lead posts and connections.
The first important change arrived in the early 1920s when a more acid-resistant, hard rubber case became de rigueur. Basic battery construction changed little during the next 30 years. Active-material performance was enhanced by use of additives (including expanders) and through improvements in raw materials.
Industrial Manufacturing Brings Advances
Increases in the efficiency of the manufacturing process were later achieved, including the introduction of machine pasting of plates. During the late 1950s, epoxy sealed one-piece covers were introduced. The case and cover material remained hard rubber, and connections between cells were still made through the cover. Lower-resistance separators made of phenolic resin-impregnated cellulose fibre came into use and significantly raised the electrical performance of cells. Battery plates stacked mechanically became common practice, reducing the manual labour, hence, lowering costs involved in lead battery manufacture.
In the early 1960s, a technique for connecting the cells within a battery in series through the cell walls was developed. Simultaneously, a method for automatically and efficiently joining plates of the same polarity within a cell element was devised. As a result, the battery’s internal resistance and the amount of connecting lead were both significantly reduced.
Battery Design Comes To The Forefront
Significant advances were also made in plate design and production techniques that gave rise to more efficient batteries with higher energy density. The late 1960s saw the introduction of injection-moulded polypropylene cases and covers providing the lead battery with a durable, thin wall, lighter weight container. The thinner outside walls and cell partitions permitted the use of more active material at no sacrifice of weight or volume of the battery. Very durable and low resistance separators became available as a final step of improvement in the flooded battery design providing for further increased battery life.
Meanwhile, a dramatic change was in the wind. The classic flooded lead-acid battery design contains the electrolyte medium as an unbounded liquid filled to a level above the top of the plates and over the busbars. Consequently, the cells must be vented to release gases (oxygen at the positive and hydrogen at the negative electrodes) liberated during charging. Not only is water lost to the venting (and thus must be replaced regularly), but the battery can be safely used only in the upright position, or spillage of the sulfuric acid electrolyte solution occurs. Also, the vented gases carry a fine mist of sulfuric acid that is highly corrosive and unhealthy in the environment.
Valve Regulated Technology Arrives
Efforts were made to develop sealed batteries not demanding topping up with water and would be safe under conditions of use which would not be abnormal for the applications in which they were deployed. These efforts culminated with the development of the valve-regulated lead-acid (VRLA) battery. The first commercial units of which were introduced by Sonnenschein in the late 1960s and by Gates Energy Products in the early 1970s.
These were the gel and absorptive glass mat (AGM) technologies, respectively. In the VRLA design, oxygen evolved during charging passes through a gas space to the negative electrode, where it is reduced (recombines) back to water. This is known as the internal oxygen-recombination cycle. There are two alternative techniques for providing the needed gas space. One design has the electrolyte immobilised as a gel within the cell; the other has the electrolyte held within AGM separators. Gas passes through clefts in the gel or through channels in the AGM separator.
Because a corresponding recombination cycle for hydrogen is not possible, and the fact that oxygen recombination is not complete (the efficiency is typically 93% to 99%), each cell is fitted with a one-way valve as a safeguard against catastrophic pressure build-up. Hence, the term valve-regulated. The VRLA battery can be employed in any orientation and is more resistant to being jostled in use.
IMAGE
7. Expander Development for Lead Acid Batteries
The use of organic expanders in lead-acid batteries has been prolific since the early 1900s. The types of organic expander used have ranged, but most variants have been wood or plant-based. Organics have ranged from wood itself and its derivatives to humics and coal precursive materials. One of the first patents mentioning the use of wood as an addition agent in negative plates is Tobiansky’s from 1899.
The high-rate discharge capacity of a lead-acid battery having plain litharge negatives is much less than the theoretical capacity, and the difference increases as the temperature of discharge decreases. The use of expanders in the negative plates makes possible the discharging of a larger percentage of the theoretical capacity of the battery. This additional capacity does not come from the expander itself, but rather that the expander has made the additional capacity available. How a small amount of expander can double or triple the useful capacity of a battery involves both chemical and physical phenomena.
Expander Principles Explained
The greater the surface area of lead particles, the greater the discharge capacity of the battery. Organic expanders adsorb onto the surface of the lead particles and through Van der Waals forces of repulsion prevent small lead particles from becoming larger crystals. An abundance of smaller lead particles leads to better discharge capacity due to greater surface area and, therefore, greater interaction with the electrolyte. This enables higher ion transfer between the negative and positive, both for discharge and re-charge.
Organic expanders in practical use are combined with barium sulfate (blanc fixe) and carbon, usually carbon black. The carbon black serves various functions, but primarily it seems to be of value in the clearing of the negative plate on formation. It may give the active material the necessary electric conductivity during the early part of formation in which its permeability to acid is at a minimum. Towards the end of formation, the carbon black appears to help remove the grey surface film occasionally encountered. Carbon black is also useful in helping to distinguish unformed negative and positive plates from each other during the manufacturing/assembly process, the negatives being darker due to the presence of the carbon black. Barium sulfate is used as it seems to stabilise the good effect of the organic over a longer period of useful life than if the barium sulfate were absent.